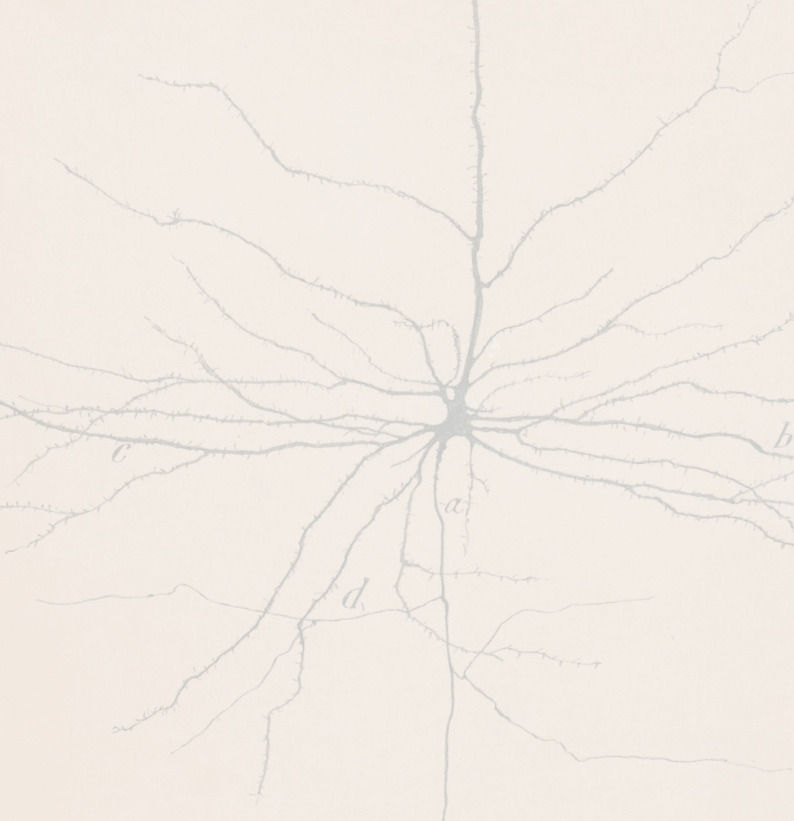
CELLULAR BIOCHEMISTRY LAB @ TAMUK
NERVE REGENERATION
1. Our objectives:
Nerve regeneration is a complex phenomenon that has interested scientists for many years. The capacity for axonal regeneration in nervous tissues is variable among vertebrate species. An intriguing difference is observed when comparing the capacity to regenerate axons in the central nervous system (CNS) versus the peripheral nervous system (PNS) of mammals. The mammalian PNS shows a well recognized ability to support axonal regeneration, which is lacking in the mammalian CNS. An important medical consequence for humans is that pathological or traumatic damage to CNS nerve fibers results in permanent loss of function. In cold-blooded vertebrates, however, the CNS shows an axon regeneration response. Fish and amphibia have been used extensively to study the biochemistry of successful regeneration in the CNS. Several proteins involved in axonal regeneration have been identified and studied in these systems. Our research interest is to further investigate the biochemistry of nerve regeneration using the fish optic nerve system. With this objective, two proteins induced during optic nerve regeneration were cloned from goldfish and they showed significant homology to mammalian CNPases (named as RICH proteins for Regeneration Induced CNPase H omologs). CNPases are myelin marker enzymes proposed to participate in membrane-cytoskeleton interactions, but their function is not well defined yet. In vitro they show phosphodiesterase activity, hydrolizing 2',3'-cyclic-nucleotide monophosphates to 2'-nucleotide monophosphate products. We demonstrated phosphodiesterase activity for RICH proteins and were able to identify amino acids critical for this enzymatic activity. We are currently studying the RICH proteins to try to understand their biochemistry and their role in nerve regeneration. A better understanding of the biochemistry of nerve regeneration is an important step for the development of novel therapies for human conditions derived from axonal damage in the CNS.
2. A More Scientific Introduction:
The central nervous system (CNS) of warm-blooded vertebrates does not support nerve regeneration, in contrast to the regenerative potential of the peripheral nervous system (PNS) (1). After injury, CNS axons degenerate resulting in permanent loss of nervous function. This phenomenon has clinical implications for humans and the study of the biochemistry involved in axonal regeneration is of considerable biomedical interest. In cold-blooded vertebrates the CNS shows marked regenerative potential. The teleost and the amphibian optic nerve have been extensively used as model systems of successful regeneration in the CNS (2,3). After injury, the axons of the retinal ganglion cells (RGCs) regenerate and reconnect with their targets in the tectum (4-6). Biochemical studies with these systems has led to the identification of several proteins that are induced in neurons that are regenerating their axons, which therefore may play a role in axonal regeneration. These proteins are known as axonal growth associated proteins (abbreviated as GAPs) and their study is of great importance to understand the regeneration process, the differences of capacities for regeneration or even for interventions aimed at improving the regeneration response They include cytoskeletal proteins (7,8), cell adhesion proteins (9,10), ion channels (11), transcription factors and other proteins of less well defined function like GAP-43 (12-14) and RICH proteins (7).
RICH proteins represent a new family of GAPs that was initially shown to be induced in regenerating retinal ganglion cells (RGCs) of goldfish. In goldfish there are two acidic proteins that were designated p68/70 upon their discovery, to reflect their apparent molecular weight (7). The protein doublet was purified from brain tissues and was shown to represent two related proteins partially associated to the plasma membrane (15). The purified proteins were used to generate partial peptide sequences that were used to clone cDNAs encoding p68/70 related proteins (16,17). Sequence analysis showed significant homology to a marker enzyme of mammalian myelin: CNPase (2',3'-cyclic-nucleotide 3'-phosphodiesterase) (18). Consequently, the encoded proteins were re-designated gRICH68 and gRICH70 (for g oldfish Regeneration Induced CNPase Homologs of 68 and 70 kDa). The recombinant proteins were expressed both in prokaryotic and eukaryotic systems and it was shown that they possess 2',3'-cyclic-nucleotide 3'-phosphodiesterase activity, identifying the gRICH proteins as novel non-mammalian members of the CNPase family (17). A highly specific polyclonal antibody was generated against recombinant gRICH and was used to confirm the identity of the two proteins with the p68/70 doublet components. The antibody was also used in immunodepletion experiments to suggest that these gRICH proteins are the major 2',3'-cyclic-nucleotide 3'-phosphodiesterases in goldfish retinas (17). A cDNA encoding a RICH protein was cloned from a zebrafish library (19). Both the corresponding mRNA and protein (designated zRICH) are induced during regeneration of the optic nerve in zebrafish. Site directed mutagenesis has identified two residues (H334, T336) in zRICH that are necessary for catalytic activity (19). The zebrafish is emerging as a model system for classic and molecular genetic studies (20,21), offering great potential for future studies aimed at discovering a role of these proteins in nerve regeneration.
References.
-
Bahr, M. and Bonhoeffer, F. (1994). Perspectives on axonal regeneration in the mammalian CNS. Trends Neurosci. 17(11):473-479.
-
Agranoff, B. W. and Ford-Holevinski, T. S. (1984). Biochemical aspects of the regenerating goldfish visual system. Axonal transport in neuronal growth and regeneration . New York, Plenum Press. 69-86.
-
Grafstein, B. (1991). The goldfish visual system as a model for the study of regeneration in the central nervous system. Development and plasticity of the visual system . London, Macmillan. 190-205.
-
Sperry, R. W. (1948). Patterning of central synapses in regeneration of the optic nerve in teleosts. Physiol. Zool. 21:351-361.
-
Attardi, D. G. and Sperry, R. W. (1963). Preferential selection of central pathways by regenerating optic fibers. Exp. Neurol. 7 :46-64.
-
Sperry, R. W. (1963). Chemoaffinity in the orderly growth of nerve fiber patterns and connections. Proc. Natl. Acad. Sci. USA 50 :703-710.
-
Heacock, A. M. and Agranoff, B. W. (1982). Protein synthesis and transport in the regenerating goldfish visual system. Neurochem. Res. 7 (6):771-788.
-
Glasgow, E., Druger, R. K., Levine, E. M., Fuchs, C. and Schechter, N. (1992). Plasticin, a novel type III neurofilament protein from goldfish retina: increased expression during optic nerve regeneration. Neuron 9 :373-381.
-
Vielmetter, J., Lottspeich, F. and Stuermer, C. A. O. (1991). The monoclonal antibody E 587 recognizes growing (new and regenerating) retinal axons in the goldfish retinotectal pathway. J. Neurosci. 11 :3581-3593.
-
Paschke, K. A., Lottspeich, F. and Stuermer, C. A. O. (1992). Neurolin, a cell surface glycoprotein on growing retinal axons in the goldfish visual system, is reexpressed during axonal regeneration. J. Cell Biol. 117 :863-875.
-
Hieber, V., Agranoff, B. W. and Goldman, D. (1992). Target-dependent regulation of retinal nicotinic acetylcholine receptor and tubulin RNAs during optic nerve regeneration in goldfish. J. Neurochem. 58 (3):1009-1015.
-
Benowitz, L. I., Shashoua, V. E. and Yoon, M. G. (1981). Specific changes in rapidly transported proteins during regeneration of the goldfish optic nerve. J. Neurosci. 1:300-307.
-
Skene, J. H. P. and Willard, M. (1981). Changes in axonally transported proteins during axon regeneration in toad retinal ganglion cells. J. Cell Biol. 89 :86-95.
-
Skene, J. H. (1989). Axonal growth-associated proteins. Annu. Rev. Neurosci. 12:127-156.
-
Leski, M. L. and Agranoff, B. W. (1994). Purification and characterization of p68/70, regeneration-associated proteins from goldfish brain. J. Neurochem. 62 (3):1182-1191.
-
Ballestero, R. P., Wilmot, G. R., Leski, M. L., Uhler, M. D. and Agranoff, B. W. (1995). Isolation of cDNA clones encoding RICH: A protein induced during goldfish optic nerve regeneration with homology to mammalian 2',3'-cyclic-nucleotide 3'-phosphodiesterases. Proc. Natl. Acad. Sci. USA 92 :8621-8625.
-
Ballestero, R. P., Wilmot, G. R., Agranoff, B. W. and Uhler, M. D. (1997). gRICH68 and gRICH70 are 2',3'-cyclic-nucleotide 3'-phosphodiesterases induced during goldfish optic nerve regeneration. J. Biol. Chem. 272 :11479-11486.
-
Sprinkle, T. J. (1989). 2',3'-Cyclic nucleotide 3'-phosphodiesterase, an oligodendrocyte-schwann cell and myelin associated enzyme of the nervous system. CRC Crit. Rev. Neurobiol. 4(3):235-401.
-
Ballestero, R. P., Dybowski, J. A., Levy, G., Agranoff, B. W. and Uhler, M. D. (1999). Cloning and characterization of zRICH, a 2',3'-cyclic-nucleotide 3'- phosphodiesterase induced during zebrafish optic nerve regeneration. J Neurochem 72 (4):1362-71.
-
Eisen, J. S. (1996). Zebrafish make a big splash. Cell 87 (6):969-977.
-
Gaiano, N. and Hopkins, N. (1996). Introducing genes into zebrafish. Biochim. Biophys. Acta 1288(1):O11-O14.
3. Our publications related to this topic:
​
-
Ballestero, R.P., Wilmot, G.R., Leski, M.L., Uhler, M.D. and Agranoff, B.W. (1995). Isolation of cDNA clones encoding RICH: A protein induced during goldfish optic nerve regeneration with homology to mammalian 2',3'-cyclic-nucleotide 3'-phosphodiesterases. Proc. Natl. Acad. Sci. USA. 92: 8621-8625
-
Ballestero, R.P., Wilmot, G.R., Agranoff, B.W. and Uhler, M.D. (1997). gRICH68 and gRICH70 are 2',3'-cyclic-nucleotide 3'-phosphodiesterases induced during goldfish optic nerve regeneration. J. Biol. Chem. 272: 11479-11486
-
Ballestero, R.P., Dybowski, J.A., Levy, G., Agranoff, B.W. and Uhler, M.D. (1999) Cloning and characterization of zRICH, a zebrafish 2',3'-cyclic-nucleotide 3'-phosphodiesterase induced during zebrafish optic nerve regeneration. J. Neurochem. 72: 1362-1371 .
-
Vancha AR, Govindaraju S, Parsa KV, Jasti M, Gonzalez-Garcia M, Ballestero RP. (2004) Use of polyethyleneimine polymer in cell culture as attachment factor and lipofection enhancer. BMC Biotechnol. 4(1):23 .
-
Challa M., Chapa G.R., Govindaraju S., Gonzalez-Garcia M. and Ballestero R.P. (2006) Characterization of the Domains of zRICH, a Protein Induced During Optic Nerve Regeneration in Zebrafish. Brain Res. Jul19; 1100 (1): 42-54 .
-
Pathi S.S., Jose S., Govindaraju S., Conde J.A., Romo H.E., Chamakura K.R., Claunch C.J., Benito-Martin A., Challa-Malladi M., Gonzalez-Garcia M., and Ballestero R.P. (2012) zRICH, a Protein Induced During Optic Nerve Regeneration in Zebrafish, Promotes Neuritogenesis and Interacts with Tubulin. Brain Res. 1474: 29-39.
-
Gelalcha, F.G., Gonzalez-Garcia M., Perez-Ballestero R., Polyethyleneimine: Cell Cultures. In: Encyclopedia of Biomedical Polymers and Polymeric Biomaterials (2014). Edited by Mishra, M., Taylor and Francis, New York. DOI: 10.1081/E-EBPP-120051076.
-
​Bandla A.C., Sheth A.S., Zarate S.M., Uskamalla S., Hager E.C., Villarreal V.A., Gonzalez-Garcia M., and Perez-Ballestero R. Enhancing Structural Plasticity of PC12 Neurons During Differentiation and Neurite Regeneration with a Catalytically Inactive Mutant Version of the zRICH Protein. BMC Neuroscience 2023, 24: 43 (pages 1-15). https://doi.org/10.1186/s12868-023-00808-1.
4. Would you like to see some figures?
​
​
​
​
​
​
​
​
​
​
​
​
​
FIG. 1. The fish optic nerve regeneration model system. Mammals cannot regenerate nerve fibers in the central nervous system (CNS), a phenomenon that has important biomedical consecuences for humans. Conversely, fish have the capacity to regenerate axons in the CNS. The optic nerve model system offers numerous advantages for anatomical and biochemical studies of nerve regeneration in the CNS. In this system, after the optic nerve is crushed, the retinal ganglion cells of the retina project regenerating axons that re-connect to the tectum (the target in the brain) in a few weeks.
​
​
​
​
​
​
​
​
​
​
​
​
FIG. 2. Time course of RICH mRNA expression during regeneration. Several axonal Growth Associated Proteins (GAPs) have been discovered with the fish optic model system. We are studying a GAP designated RICH (Regeneration Induced CNPase Homolog), which is highly induced in the regenerating optic nerve. The figure shows the induction of the RICH mRNA during regeneration by RNA protection assay. Sense positive controls were performed with the indicated amounts (pg) of sense RNA. The number of days post-crush is indicated above; C indicates that the RNA was extracted from the control retina (no nerve crush); R indicates that the RNA was extracted from the regenerating retina. Four µg of total RNA were used per lane. C0 is from control fish that did not receive optic nerve crush. The positions for the g-RICH and 28S rRNA (internal control) protected fragments are indicated on the left. Notice the induction of the g-RICH mRNA fragment in the regenerating retinas compared to the normal retinas. The induction is maximal (8-fold) 20 days after the nerve crush.
​
​
​
​
​
​
​
​
​
​
​
​
​
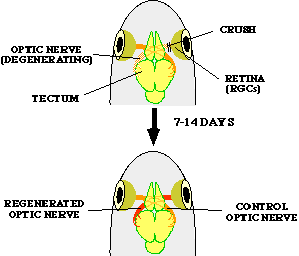
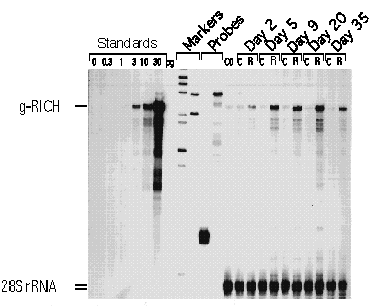
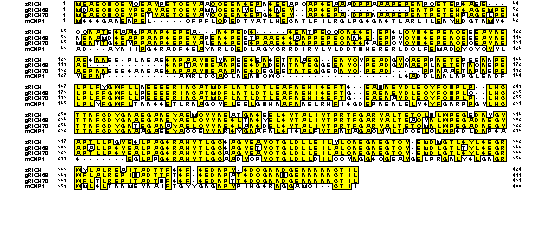
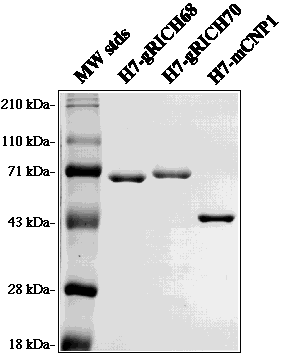
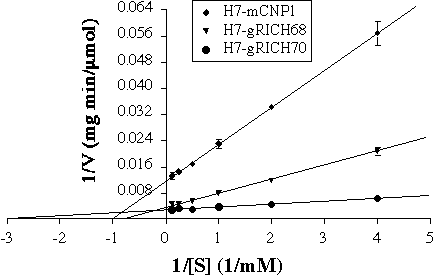
FIG. 3. Alignment of zRICH gRICH68, gRICH70 and mCNP1. We have been able to clone two RICH proteins from goldfish, gRICH68 and gRICH70, and one RICH protein from zebrafish, zRICH. The sequence of all the RICH proteins was aligned by the Clustal method. The sequence of a mammalian CNPase enzyme (mCNP1) was included in the alignment for comparison. Note the high degree of amino acid identity among the RICH proteins. The highest divergence is localized to the N-terminal one third of the molecule, the region of lowest homology with mammalian CNPases. The putative catalytic domain (C-terminal two thirds) is very conserved among all the RICH proteins and shows high homology to mCNP1, including an isoprenylation motif at the C-terminus.
​
​
​
​
​
​
​
​
​
​
​
​
FIG. 4. Expression and purification of recombinant CNPases in E. coli. gRICH68, gRICH70 and mCNP1 were expressed in E. coli as heptahistidine-tagged proteins. The recombinant proteins were purified to near-homogeneity by nickel-NTA affinity chromatography. Five µg of each protein were run in a 10% SDS-PAGE and the gel was stained with Coomassie brilliant blue. Molecular weight marker sizes are indicated on the left. Note the abnormal migration of the gRICH proteins, approximately 20-25 kDa above their predicted molecular weight.
​
​
​
​
​
​
​
​
​
​
​
​
​
FIG. 5. Enzymatic characterization of gRICH proteins. The purified proteins were used in CNPase assays with 2',3'-cAMP as substrate by a coupled-assay colorimetric method. The initial velocities determined at several substrate concentrations were used to generate Lineweaver-Burk plots. The plots show the average +/- SD of triplicate measurements and is representative of three independent determinations for each enzyme. Error bars not showing were contained within the symbol. Km and Vmax parameters were determined from the plots for each enzyme.
​
​